The central nervous system consists of more than 80 billion nerve cells (“neurons”). These neurons are networked with each other and connected to other parts of the body by “axons,” the long projection that extends from the neuron to its target tissues. The spinal cord contains both bundles of these axons, carrying information between the brain and the periphery, and relay centers, where signals are analyzed, filtered, and amplified. The brain receives sensory information about the world. It controls the motor activities of the body using signals sent through the long axons, many of which travel in the white matter of the spinal cord. When the spinal cord is damaged or severed, the connections between nerve cells in the brain and their targets in the periphery are disrupted. The nerve fiber that is distal to the injury undergoes degeneration. Even if the nerve cell body attempts to heal by sprouting new fibers, there is no way to carry the message to the target tissue once the distal axon is gone. For many years, the prevailing view was that the nerve’s axon dies much like a flower dies if its stem is severed. The ‘vital juices’ seep out. In recent years, it was realized that this image of damage to distal (or ‘downstream’) axons is wrong. After a cord injury, the distal axon is still nourished and supported by surrounding cells. The axon downstream of an injury degenerates because an active signal is sent to it, causing its breakdown. Some believe this ‘self-destruct’ signal serves the purpose of ‘decluttering’ the spinal cord. Regardless, the process of axonal death after a spinal cord injury makes it much more difficult for the body to reestablish functional connections across the gap in the spinal cord created by the initial injury.
About a decade ago, Dr. Strittmatter and his colleagues identified a group of molecules ordinarily present in the spinal cord that limit the ability of nerve fibers to grow and regenerate. 1 Some of the specific molecules that inhibit neuronal regrowth are called Nogo-A, MAG, and OMgp, and they exert their effects (inhibiting repair) by binding to a specific receptor (NgR1). Dr. Strittmatter and others then set out to find ways to block the effects of these molecules, which inhibit the regrowth and reconnection of axons. His team created a new drug, a molecule (NgR1-FC, also known as AXER-204) that binds to these inhibitory molecules. 2 The new drug acts as a decoy; the inhibitory substances bind to the drug rather than bind to the NgR1 receptor, whose activation is limiting the ability of surviving neurons to sprout axons and reorganize their connections. The new drug has recently been proven safe and effective in animals (including primates) with spinal cord injuries. Importantly, this drug had an effect in animal models long after the initial injury, indicating that the innate capability of neurons to regenerate connections and functions persists long after an injury.
The preclinical data was sufficiently encouraging that the RESET clinical trial was launched in humans. 3 It is anticipated that the results of this trial will be available this year. A similar phase 2 clinical trial is underway in Europe; its results are also anticipated shortly. Positive results in either of these trials would be a true breakthrough in the quest to stimulate brain regeneration. Such results would demonstrate that neurons have an innate ability to self-repair that is normally inhibited but can be reactivated after a severe injury. There is good reason to believe that the same or similar mechanisms that inhibit neuronal recovery in the spinal cord are also active in the brain after a brain injury. 4 The DLF is following this line of research with great interest and enthusiasm. The ability to unlock the innate power of a damaged neuron to regrow and reconnect is one critical step toward brain regeneration and functional recovery after a major brain injury.
References
- Schwab, M. E. & Strittmatter, S. M. Nogo limits neural plasticity and recovery from injury. Curr. Opin. Neurobiol.27, 53–60 (2014).
- Wang, X. et al. Nogo receptor decoy promotes recovery and corticospinal growth in non-human primate spinal cord injury. Brain143, 1697–1713 (2020).
- AXER-204 in Participants With Chronic Spinal Cord Injury - Full Text View - ClinicalTrials.Gov. https://clinicaltrials.gov/ct2/show/NCT03989440.
- Lindborg, J. A. et al. Optic nerve regeneration screen identifies multiple genes restricting adult neural repair. Cell Rep.34, 108777 (2021).
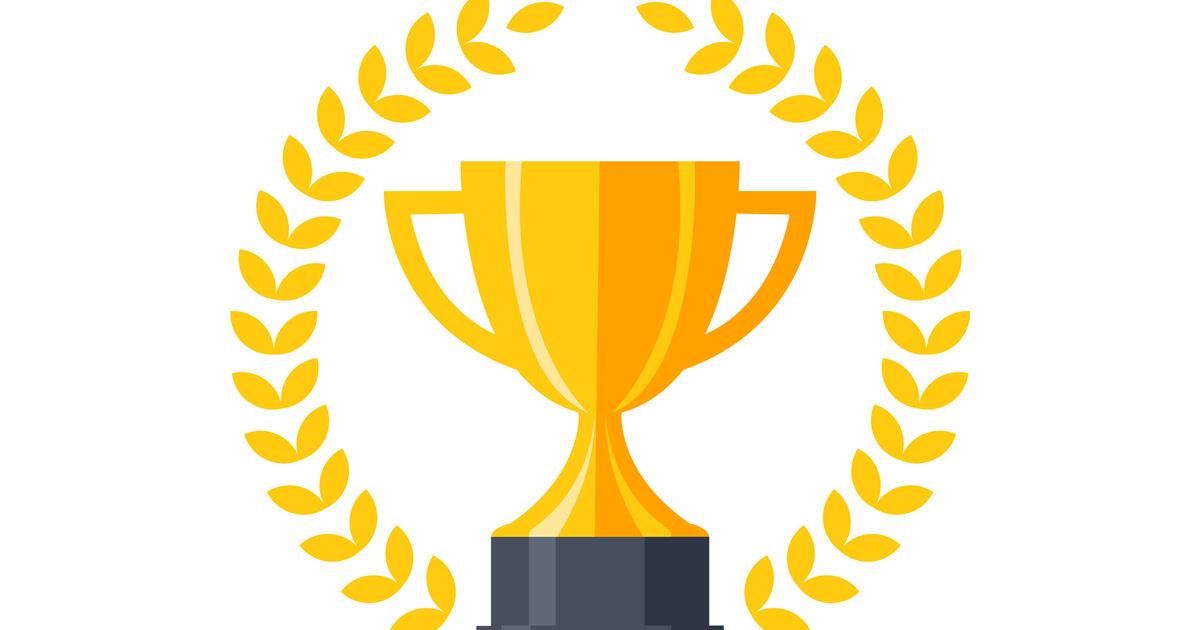
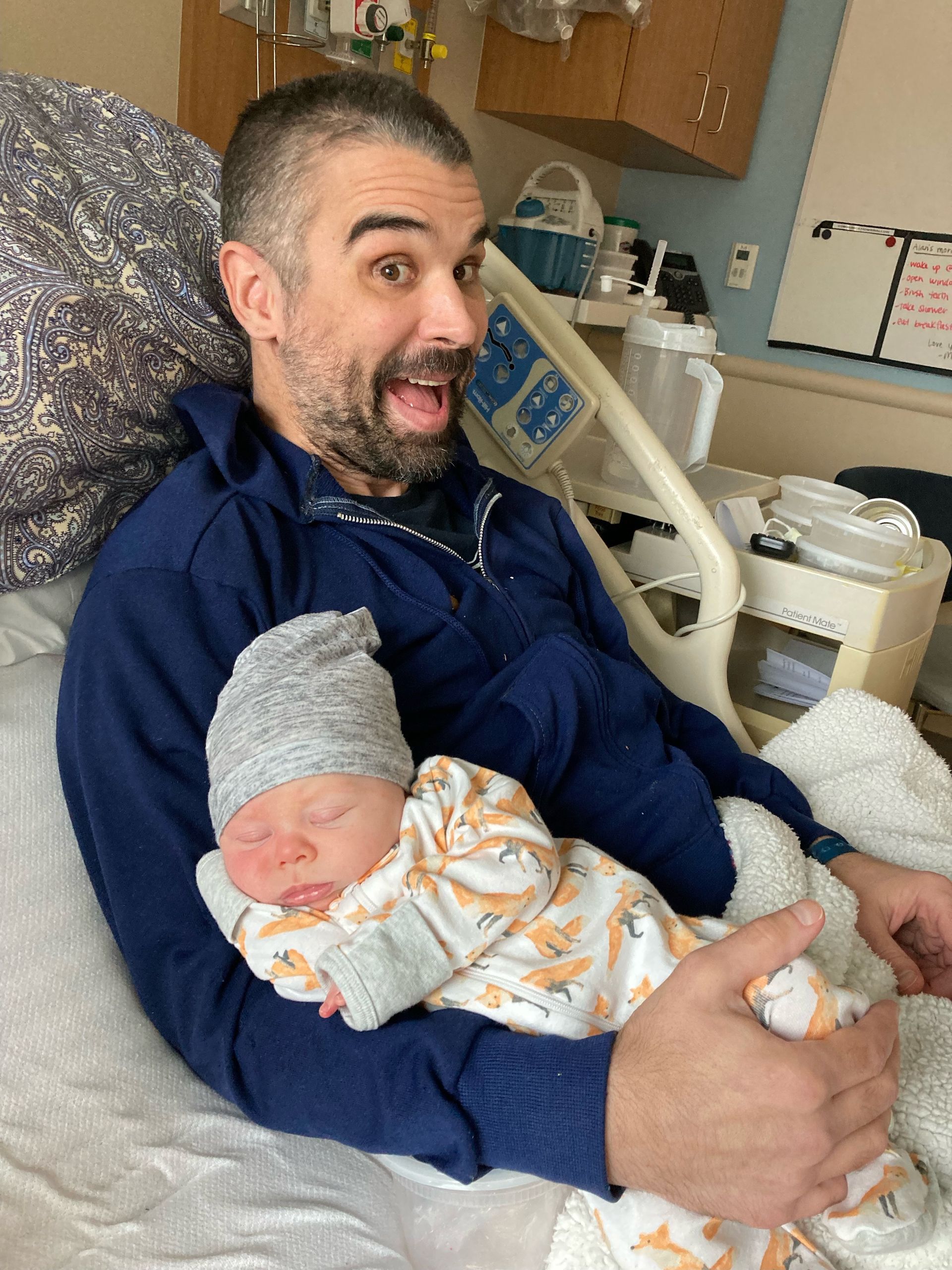