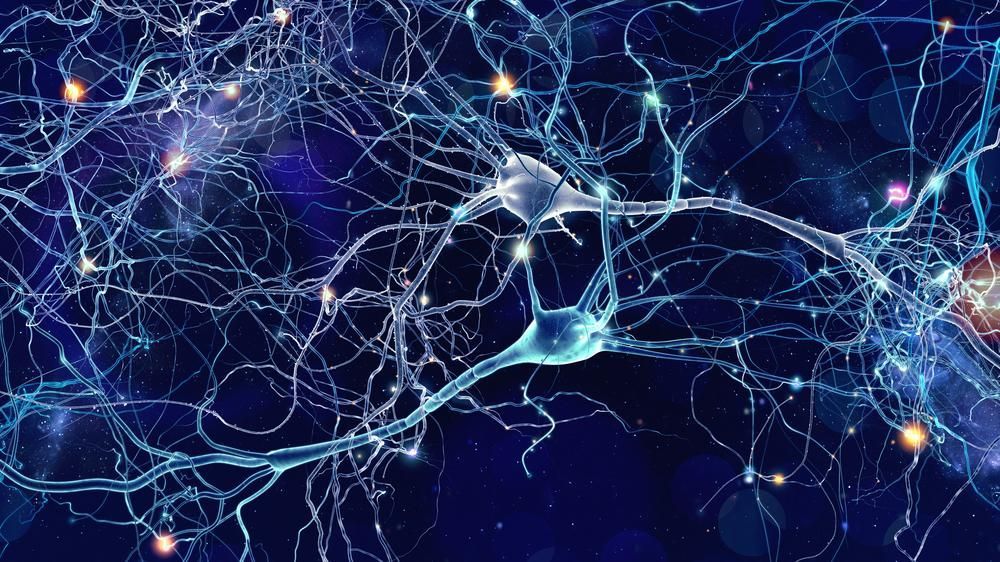
Introduction: The Paradigm Shift in Brain Regeneration
For decades, scientists believed that the adult brain was incapable of meaningful regeneration. Unlike skin or muscle, the central nervous system (CNS) appeared to lack the ability to repair itself after injury, and lost neurons were considered irreplaceable. However, cutting-edge research now demonstrates that this is no longer the case. The brain possesses dormant repair mechanisms—pathways that were active during development but have been shut down in adulthood. By reactivating these pathways, it may be possible to induce neurogenesis (the birth of new neurons), axonal repair, and synaptogenesis (the formation of new neuronal connections) after devastating injuries like stroke, traumatic brain injury, and spinal cord damage.
A growing body of evidence from both the spinal cord and the central nervous system (the CNS) shows that regeneration can be stimulated by downregulating the repressing factors that prevent neuron growth and repair. For clarity, “downregulation” means reducing the activity or number of something. In the case of a repressing factor, downregulation means lowering its levels or making it less active. A repressor is a protein that blocks a process—often by preventing a gene from being turned on. When the repressor is downregulated, it no longer effectively blocks that process, allowing the underlying function to be released or activated.
These advances open the door to new treatments that could restore function to patients with neurological injuries, potentially reversing what were once thought to be permanent disabilities.
The Science Behind Reactivating Brain Repair
Neurodevelopmental genes and pathways that promote neuronal growth and plasticity are switched off after early development. However, researchers have identified key molecular regulators that act as “brakes” on brain regeneration. The “brakes” act as repressors of brain regeneration. By inhibiting these repressors, the brain’s intrinsic ability to regrow neurons and axons can be reactivated.
Recent breakthroughs include:
- Neurogenesis in the Adult Brain: Research has shown that downregulation of PTBP1, an RNA-binding protein, can convert glial cells into neurons in the adult brain. Studies using antisense oligonucleotide (ASO) therapy to transiently suppress PTBP1 have successfully induced the formation of functional neurons in mouse models of neurodegeneration. For clarity, an antisense oligonucleotide (ASO) is a short strand of DNA or RNA designed to bind to a specific mRNA and regulate gene expression. By binding to its target, an ASO can block protein production, modify splicing, or mark the mRNA for destruction, effectively acting as a precise “off switch” for genes. In this context ASOs can be used to release glial cells to develop into functional neurons. Work is now underway to create ASOs that can be safely administered to humans, thereby stimulating the creation of new neurons.
- Axonal Regeneration in the CNS: Another major discovery involves unlocking nerve fiber regrowth by downregulating specific nerve growth inhibition, which prevents nerve fiber regrowth. Studies have demonstrated that suppressing these inhibitors leads to long-distance axonal regeneration, restoring function in spinal cord injury models.
- Synaptic Repair in Neurodegenerative Disease: Scientists have shown that modulating key synaptic receptors—such as mGluR5—can restore lost synaptic connectivity, improving brain network function in neurodegenerative diseases like Alzheimer’s. The neuronal connections and circuits in the brain are maintained in a stable state by the balanced action of different synaptic receptors. By modifying this balance, the rate of brain synapse formation can be increased or decreased.
Together, these findings confirm that neural repair is possible when the appropriate repressive factors are removed, unlocking the brain’s natural regenerative capacity.
Quiver’s Scalable Human Neuronal Platform: Accelerating Discovery
While these discoveries are promising, translating them into effective therapies requires precise, scalable, and high-throughput screening technologies that can screen out or discover biomolecules that address a specific biomedical target by the thousands with great rapidity. One such technology has been developed by Quiver Biosciences using an all-optical electrophysiology platform. (Note: the author of this article is a co-founder of Quiver Biosciences)
Quiver has developed a human induced pluripotent stem cell (hIPSC)-derived neuronal platform that allows researchers to measure functional activity in human neurons at an unprecedented scale. This platform is uniquely capable of:
- Directly measuring neuronal excitability, synaptic transmission, and network connectivity using optogenetics and advanced imaging techniques.
- Screening for new factors that inhibit brain repair, helping scientists identify additional repressors that need to be targeted.
- Evaluating small molecules and ASO-based therapies that may upregulate brain regeneration, assessing their efficacy and toxicity before advancing to clinical trials.
The potential of this platform is vast. Scientists can now systematically search for drugs that mimic the effects of PTBP1 suppression, Nogo-A blockade, or mGluR5modulation—treatments that could one day be used to regrow neurons, reconnect severed axons, and restore lost synapses in patients with brain injuries.
Key advantages of Quiver’s platform include:
- Scalability: Unlike traditional animal models, this system enables high- throughput drug screening on human neurons, increasing the efficiency of therapeutic discovery.
- Relevance: Because the neurons are derived from human stem cells, they offer a more accurate model of human brain function and disease than animal-based approaches.
- Safety Screening: The platform allows for early identification of toxic effects, ensuring that only the safest and most effective compounds move forward in development.
By integrating AI and machine learning, Quiver’s platform also enables pattern recognition of successful drug candidates, identifying compounds with optimal efficacy and minimal side effects.
The Role of Philanthropy: Funding the Future of Brain Repair
Scientific breakthroughs alone are not enough to bring regenerative therapies to patients. Translational research—the process of developing basic discoveries into real-world treatments—is slow, expensive, and underfunded. This is where philanthropic support can make an immediate impact.
Many of the pioneering studies on brain regeneration were initially considered too risky or unconventional for traditional funding sources. Yet, thanks to early philanthropic investments, these ideas have now been validated and are shaping the next generation of treatments. Today, funding is urgently needed to:
- Expand screening for regeneration-promoting drugs
- Optimize ASO-based approaches for inducing neurogenesis and axonal repair.
- Accelerate clinical trials for promising regenerative therapies.
A New Era for Brain Regeneration
For the first time, we have the tools to reactivate the brain’s own repair mechanisms, offering hope for individuals with severe neurological injuries. The path forward is clear: by investing in innovative research, scaling up discovery efforts, and supporting translational studies, we can bring brain-regenerating therapies to patients faster.
The DLF raises funds and uses them to inspire, catalyze, and accelerate work towards brain regeneration. We increase public awareness of possibilities in brain regeneration through our social media, news “blasts”, and quarterly newsletter. And, we promote neuroscientific advances through consultation, networking, conferences, and seed grants. This is especially important when federal funding is limited. By supporting this work, we can help transform groundbreaking scientific insights into real treatments that restore lost function and improve lives.
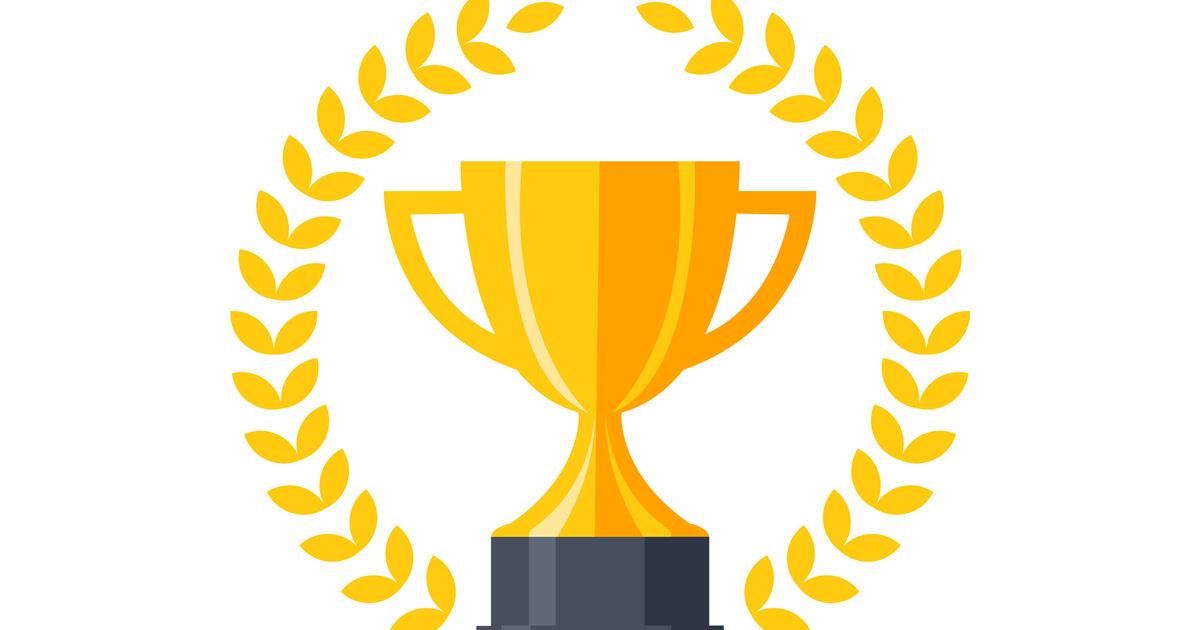
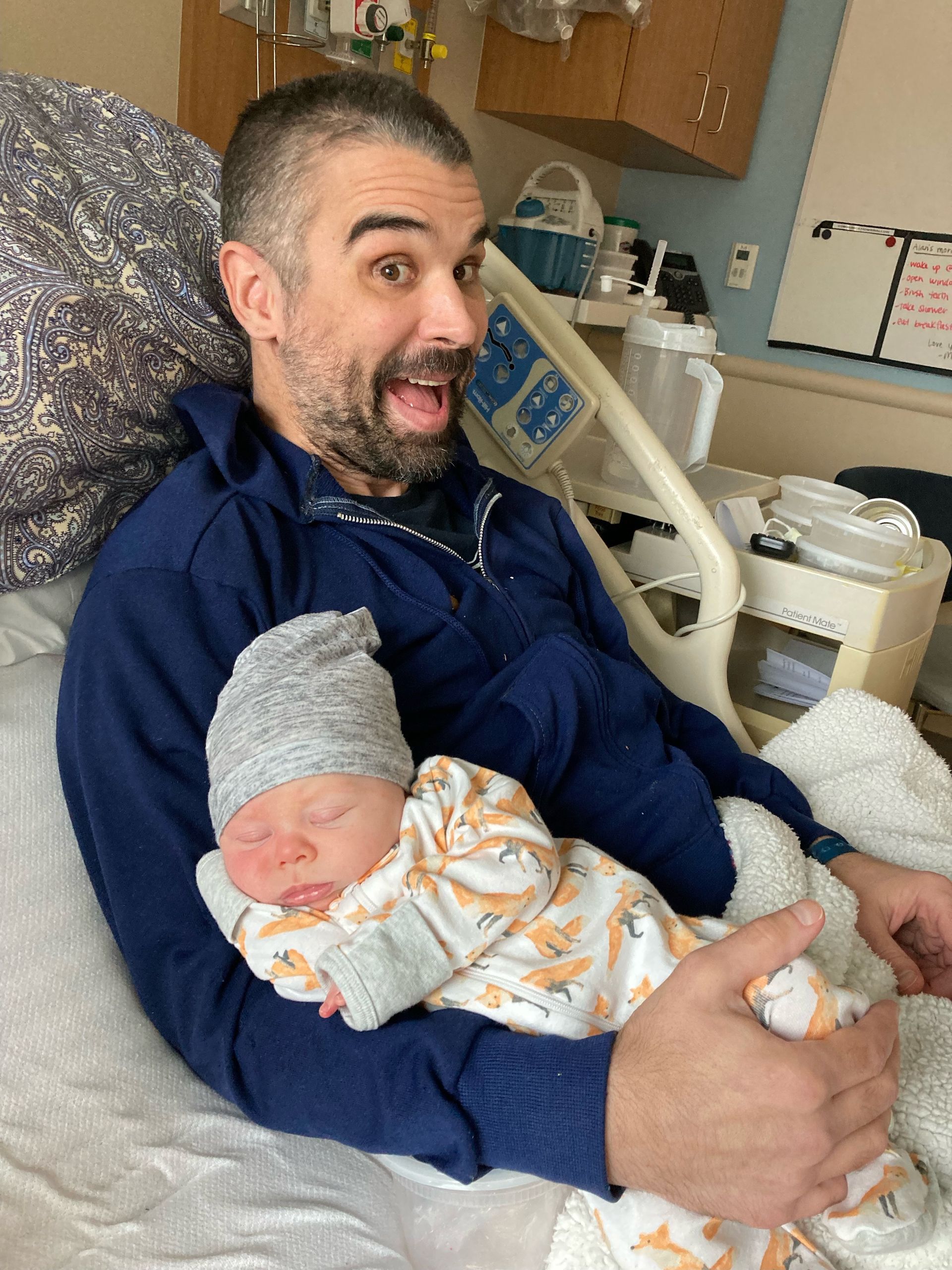