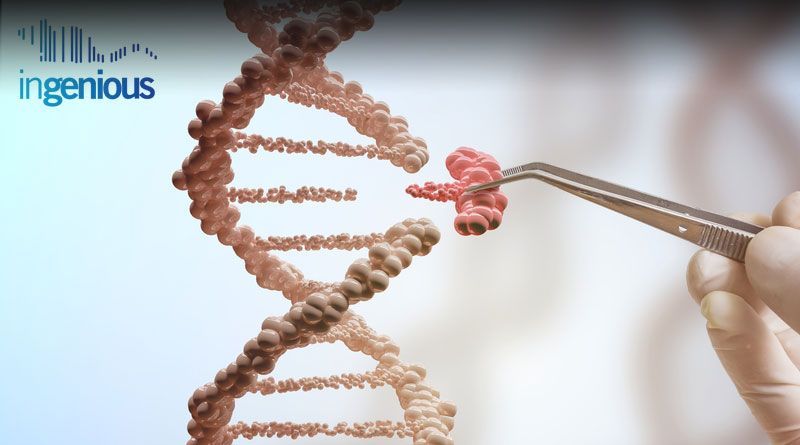
The human genome is the maestro of the brain’s formation, growth, and maturation. As a person develops and interacts with environmental stimuli, the genetic program in all cells gradually unfurls itself. In a beautifully coordinated process, our DNA transcribes its information into RNA, which in turn mediates the synthesis of proteins that are essential for all life functions. For axons to repair themselves, for new synapses to form, and for neurons to proliferate, the respective elements of the genome must spring into action. As the brain matures, some of the properties of the developing brain are lost or diminished. Stimulating a brain to regenerate after injury will require reactivating these dormant genomic functions.
How, then, have scientists focused explicitly on the challenge of stimulating brain regeneration at the genetic level?
One method, gene therapy, involves introducing new genetic material into adult cells. Gene therapy uses harmless viruses, specifically adeno-associated viruses (“AAVs”) to deliver new genetic material into cells and, thereby, modify cellular activities. This method may be advantageous to target the brain since the blood-brain barrier often prevents other small molecule therapeutics from accessing brain tissue. Many investigators are studying the use of AAVs in preclinical studies to deliver genes promoting neuronal survival and growth in models of neurodegenerative disease.¹ Gene therapy results in a permanent change in the cell’s genome. New genetic material is incorporated into the cell’s genome, and this new genetic information then controls the activity of the cells by creating new or modified proteins. By causing the synthesis of proteins required for the formation of new cells or connections, AAV-mediated gene therapy may, someday, drive meaningful brain regeneration.
Gene therapy is technically very challenging. Figuring out the right dose of virus to administer is difficult, and it is usually not possible to administer multiple doses, since the body develops an immune response to the virus. Notwithstanding the difficulties in this approach, one team has used a gene therapy construct to regenerate many functional new neurons in an adult mouse model after ischemic injury.² This study provides some evidence that there is a reservoir of cells in the brain that can potentially be converted into replacement neurons.
Another approach to modifying the genome is to use “gene editing.” In this approach, a molecular ‘cut-and-paste’ tool has been discovered and developed to insert specific corrections into the target genome. For example, researchers have initiated clinical trials using gene editing (“CRISPR-Cas9 gene editing”) to treat a genetic form of blindness, Leber’s Congenital Amaurosis.³ This form of gene editing has been performed on cells in the back of the eye, and the induced corrections have led to the partial restoration of vision. This groundbreaking achievement offers renewed hope for applying similar strategies in brain regeneration. However, it is more difficult to imagine how gene editing techniques can deliver their corrective ‘payload’ to targeted regions.
The spotlight is shifting towards a third approach to manipulating the genome by using small, DNA-like molecules called “antisense oligonucleotides (ASOs)” to modulate gene activity. Antisense oligonucleotides (ASOs) are short, synthetic strands of modified DNA that can bind to specific RNA molecules and alter their activity. An ASO is a small molecular ‘patch’ that finds a specific location in the RNA message created by the genome to guide the formation of proteins. By ‘patching’ the mRNA, an ASO either prevents the production of harmful proteins or increases the production of beneficial ones. This class of molecules can target and modulate the formation of proteins with exquisite specificity. ASOs may be useful to stimulate brain regeneration by silencing genes that inhibit neuronal growth and plasticity or boosting genes that promote these processes. ASOs can be developed relatively rapidly, and their dosage is easier to titrate compared to AAV gene therapy or gene editing approaches.
There are already several examples of ASOs in use to target genetic controls in the brain in a selective manner. For instance, the FDA-approved drug Nusinersen for spinal muscular atrophy (SMA) is an ASO that increases the production of a critical motor neuron protein, thereby improving motor function in affected children.⁴ After many years of research, clinical trials are now underway to treat Huntington’s disease with ASOs.⁵ Finally, ASOs are showing promise in early-stage clinical trials for amyotrophic lateral sclerosis (ALS) by reducing the levels of a harmful protein that accumulates in the brain cells of patients.⁶ Dozens of ASO drug development programs that target the brain are now underway. Scientists, families of afflicted patients, and biotech companies are fully engaged in promising collaborations to unlock the brain’s capacity for healing. In previous newsletters, we have identified several promising areas of research that aim to stimulate significant brain regeneration and functional recovery after a major brain injury. We’ve explored several of these: how nerve cells (neurons) can be induced to repair their long tract connections, ‘axonal repair’ after spinal cord injury⁷; how the growth of new connections between neurons in surviving brain regions can be stimulated, ‘synaptogenesis and induced plasticity’⁸; and how to stimulate the creation of new neurons, ‘neurogenesis’ or to integrate newly transplanted (replacement) cells in the brain simulating neurogenesis.⁹
These strategies all rely on a thorough understanding of how the human genome controls axonal repair, synaptogenesis, and neurogenesis. The same genomic information that guides the brain’s growth and development contains the information necessary to regenerate after damage. This generation of brain scientists is inexorably unlocking that potential and learning how to allow the brain to heal itself.
The Dan Lewis Foundation is closely following multiple lines of research in the field of brain regeneration. The DLF is committed to encouraging and catalyzing such research through collegial exchange, linking researchers, disseminating new research findings, awarding the DLF Prize, and directly funding research as funds become available.
References
1. Ozlu, C., Bailey, R. M., Sinnett, S. & Goodspeed, K. D. Gene Transfer Therapy for Neurodevelopmental Disorders. Dev. Neurosci. 43, 230–240 (2021).
2. Chen, Y.-C.et al. A NeuroD1 AAV-Based Gene Therapy for Functional Brain Repair after Ischemic Injury through In Vivo Astrocyte-to-Neuron Conversion. Mol. Ther. 28, 217–234 (2020).
3. Daich Varela, M., Cabral de Guimaraes, T. A., Georgiou, M. & Michaelides, M. Leber congenital amaurosis/early-onset severe retinal dystrophy: current management and clinical trials. Br. J. Ophthalmol.106, 445–451 (2022).
4. Finkel, R. S.et al. Nusinersen versus Sham Control in Infantile-Onset Spinal Muscular Atrophy. N. Engl. J. Med. 377, 1723–1732 (2017).
5. Rook, M. E. & Southwell, A. L. Antisense Oligonucleotide Therapy: From Design to the Huntington Disease Clinic. BioDrugs 36, 105–119 (2022).
6. Boros, B. D., Schoch, K. M., Kreple, C. J. & Miller, T. M. Antisense Oligonucleotides for the Study and Treatment of ALS. Neurotherapeutics 19, 1145–1158 (2022).
7. Towards Brain Regeneration and Functional Recovery. Making Headway: DLF NeuroConnections (Fall 2022).
8. The Synapse and Brain Regeneration. Making Headway: DLF NeuroConnections (Winter 2023).
9. Can Damaged Tissue Be Replaced? Making Headway: DLF NeuroConnections (2023).
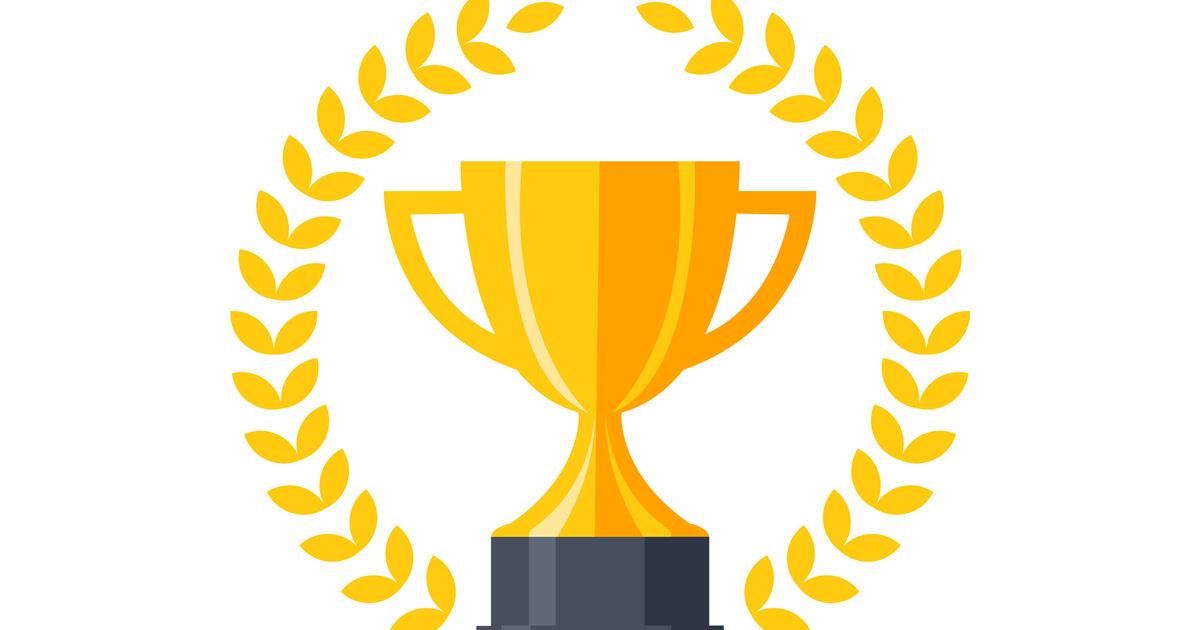
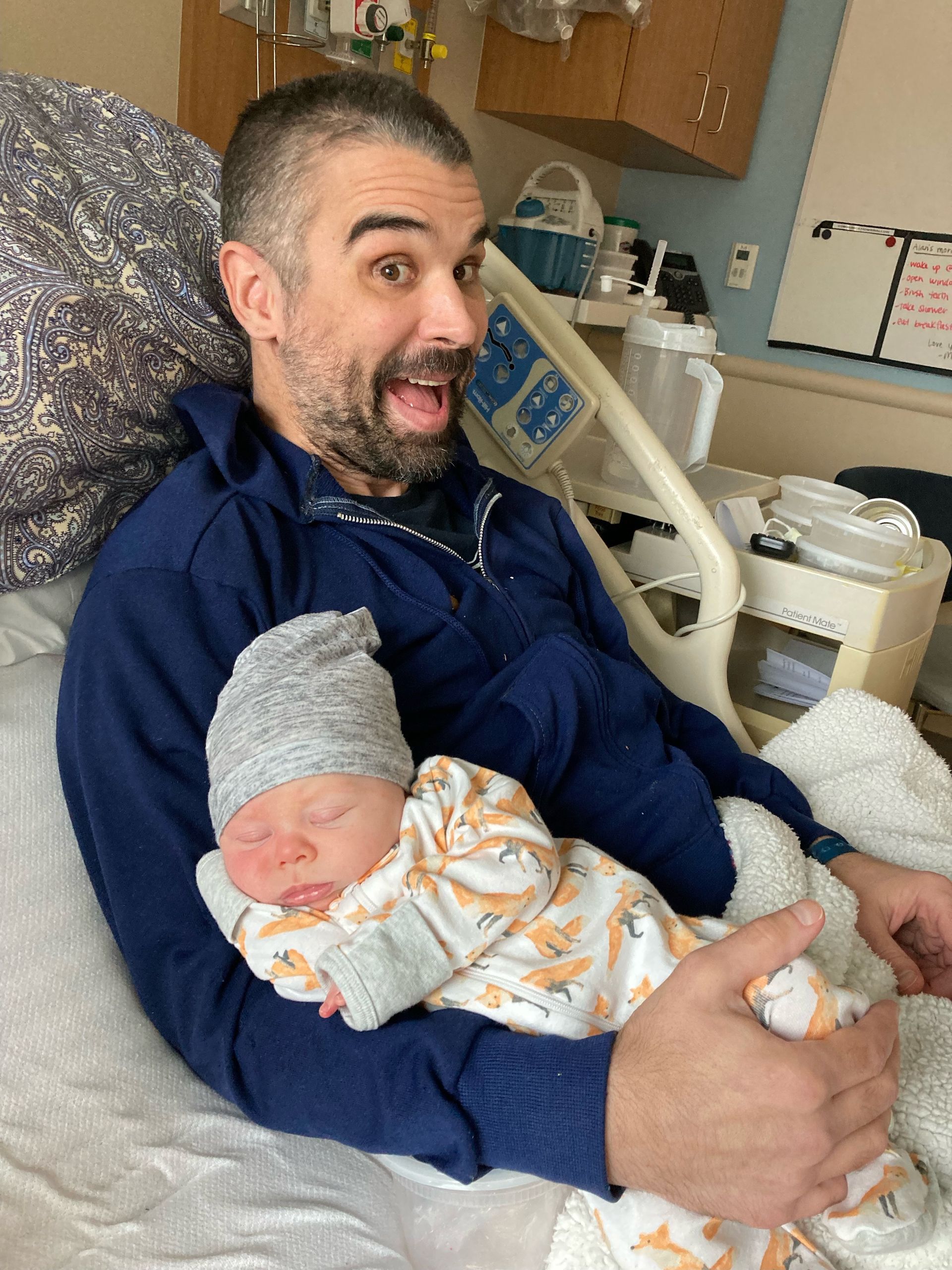