Overview of TBI What is TBI?
- Basic acute-phase physiology
- Chronic-phase physiology and natural history
Traumatic brain injuries are common, often devastating, and, for many, poorly responsive to treatment. While methods to evaluate TBI advanced substantially during recent decades and principles of supportive care have also progressed, there are no pharmacologic therapies that seek to specifically stimulate neurogenesis (growth of new neurons) or synaptogenesis during the post-acute phase of care.
The sequelae of TBI depend on the extent, nature, and location of the initial injuries, on acute phase pathophysiologic changes, and on long term rehabilitation efforts. A number of factors interact to determine the nature of the chronic deficits from a TBI, including disruption of key structures at the site(s) of injury, residual scar formation, post-traumatic electrophysiologic abnormalities, and emergent neuropsychological states.
There is a large and growing research effort to develop TBI diagnostics (Redell et al. 2010), to optimize care during the acute phase of a brain injury (Vella, Crandall, and Patel 2017), and to enhance functional recovery using neuromodulation (Hofer and Schwab 2019).
- Regulation of neurogenesis and synaptogenesis in humans (Aimone et al. 2014)
- Neurogenesis
- Pre-natal: https://en.wikipedia.org/wiki/Neurogenesis
- Perinatal:
- Hippocampal: (Yang et al. 2014)
- Adult: https://en.wikipedia.org/wiki/Adult_neurogenesis
- Synaptogenesis (Gatto and Broadie 2010)
- Regulatory factors
- Neurotrophic factors: (Cacialli and Lucini 2019) (Huang and Reichardt 2001) https://www.sciencedirect.com/topics/neuroscience/neurotrophic-factors
- Autocrines: (Herrmann and Broihier 2018)
- Cortical plasticity: (“Evolution and Ontogenetic Development of Cortical Structures” 2019; El-Boustani et al. 2018)
- Tissue regeneration in humans and animal models
- non-CNS (https://en.wikipedia.org/wiki/Regeneration_in_humans)
- non-Human CNS (Ghosh and Hui 2016) (Cacialli and Lucini 2019) (Zambusi and Ninkovic 2020)
- Human CNS
- Regulation, dysregulation, and controlled regulation (Tsintou, Dalamagkas, and Makris 2020) (Modo 2019)
The brain’s limited ability to regenerate its cells and tissue structures is a fundamental obstacle to healing in TBI. Tissue regeneration in adult humans is limited in a number of tissues while present in other tissues. Certain structures whose spatial organization is critical to function can regenerate (e.g., liver, bone (partially)). Other structures whose spatial organization is the basis of the tissue’s physiologic function are not naturally regenerated (e.g., lung, heart, brain). (Wikipedia contributors 2020)
Presumptively, two of the critical limitations on long term recovery from TBI are the loss of cells, especially cortical cells, from the injured brain regions, and the disruption of the functional connections (tracts and synapses) in the region of injury. [ ]
One observation is that all brain regions are not equivalent with regards to the retention of the capacity to form new neurons and synapses in adulthood. Both DG and olfactory bulb have active neurogenesis ((Weston and Sun 2018) in certain adult animal models, but the extent of this phenomenon in humans is unclear (Bhardwaj et al. 2006)
Modest advances have been made at inducing regeneration in human tissue that is not naturally regenerated using both tissue engineering techniques and by altering growth factors. (Modo 2019)
- Pharmacologic therapies: past efforts and trials (Diaz-Arrastia et al. 2014)
- Stem cell therapy (Weston and Sun 2018)
- Autologous embryonic stem cells
- In non-human models
- In humans (Schepici et al. 2020)
- Induced pleuripotent cells: (Omole and Fakoya 2018) (Dunkerson et al. 2014)
- Role of the bioscaffold: (Modo 2019)
Unsurprisingly, as our understanding of stem cell biology has progressed in recent years, some are attempting to replete the CNS by providing it with specially engineered stem cells. The broad concept has been reviewed (Weston and Sun 2018)
Some have claimed that stem cells can repair TBI (c.f. https://www.pacificneuroscienceinstitute.org/blog/brain-trauma/can-stem-cells-repair-traumatic-brain-injury/, but study results from an earlier trial using the same cells for patients who had suffered from an ischemic stroke were recently posted (https://clinicaltrials.gov/ct2/show/results/NCT02448641). These initial trials have not yet demonstrated any meaningful level of recovery in post-stroke patients.
- Development of a TBI therapy
- Need for model organisms: (Shah, Gurdziel, and Ruden 2019)
- Animal models
- Novel mouse models:(Reimann et al. 2019);(Chang et al. 2018)
- Tissue models
- Cellular models: (https://www.researchgate.net/profile/Ashwin_Kumaria/publication/320692835_In_vitro_models_as_a_platform_to_investigate_traumatic_brain_injury/links/5bb07ca092851ca9ed30dd12/In-vitro-models-as-a-platform-to-investigate-traumatic-brain-injury.pdf)
- Enabling model components (iPSCs; assays; analytics)
- Enabling pharmacology (genomically targeting molecules)
- Small molecules
- ASOs and other mRNA-targeting compounds (Karaki, Paris, and Rocchi 2019) (Rinaldi and Wood 2018) (definitive text: https://www.springer.com/gp/book/9781592595853) (Schoch and Miller 2017)
- A target??
- LYNX1 (Morishita et al. 2010; Miwa, Anderson, and Hoffman 2019; Higley and Strittmatter 2010; Bukhari et al. 2015; Sajo, Ellis-Davies, and Morishita 2016)
- Overview LYNX1 overview (A. Cohen, 12/20/17)
- Recent analogous efforts
- Spinal muscular atrophy (https://smanewstoday.com/spinraza-nusinersen-ionis-smnrx/)
- “Milasen” (Kim et al. 2019)
- “Lukesen” [Q-State Biosciences -- Kopin presentation in today’s meeting]
- Current efforts in this area
- ReNetX Bio: https://www.renetx.com/
- Q-State Bio: (Q-State’s platform)
- Cells: iPSCs → various types of neurons, including excitatory and inhibitory cortical neurons (Molnár et al. 2019); (McCaughey-Chapman and Connor 2018) and astrocytes (Barbar et al. 2020)
- Assays and analytics: (Williams et al. 2019)
- Specific disease models:
- Cell culture of various human and non-human primary and derived neurons with both excitation and synaptic assays
- Engineered isogenic controls
- “Slice” preparations of mice
- Therapeutic development capabilities
- New abilities to study cortex and cortical neurons using optogenetic tools (https://spaces.hightail.com/receive/YH79qaNhlU/fi-10048c4d-dbab-422c-ad37-847578ceaae0/fv-b28c8b5f-38c4-4324-9c31-c33cc19b0a2d/20200116_Adam_Cohen-Sensory_Information_Processing_1.mp4) (Fan et al. 2020) and the Q-State synaptic assays
- Others?
- https://www.agexinc.com/company-overview-biotechnology-for-gerontology-tissue-regeneration/
- https://gmpnews.net/2020/01/a-russian-drug-gets-alzheimers-patients-to-recover-the-memory/
- Antisense Oligonucleotides
- ASOs and TBI: (Shohami et al. 2000) (Fluiter et al. 2014)
- A plan?
- Targeting LYNX1 with ASOs?
Combining LYNX1 downregulation with autologous iPSC stem cell therapy?
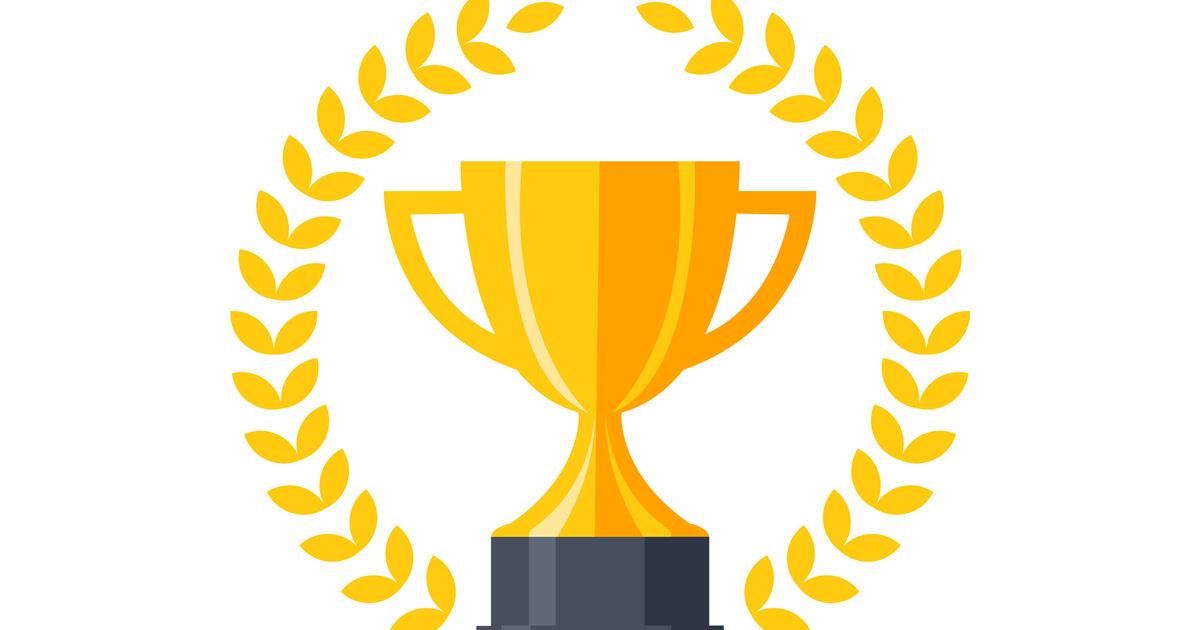
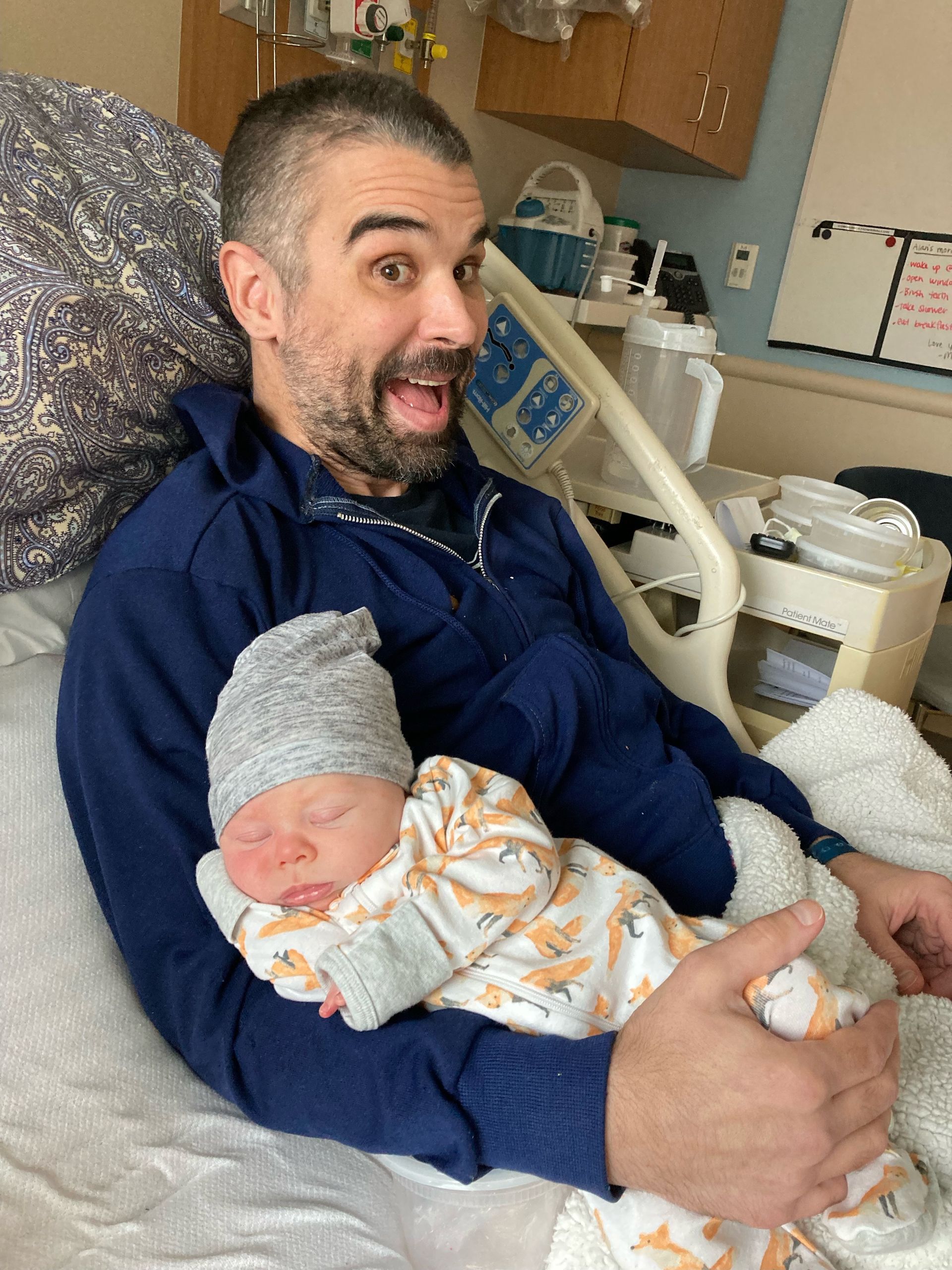