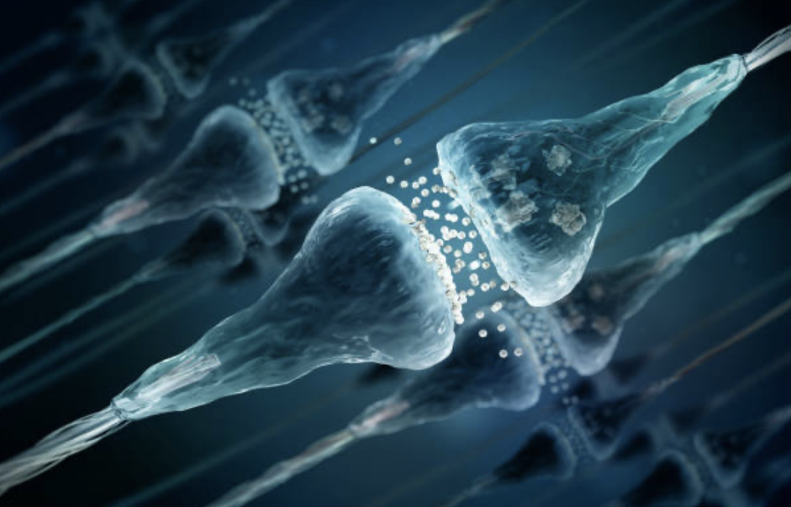
The past decade has brought new hope that the damaged brain can heal itself. It is now within the realm of possibility that scientists will design medicines that promote brain regeneration, even long after a severe brain injury. These new medicines will be engineered to use the brain’s own genetic machinery to stimulate regeneration and functional recovery. In the last edition of the DLF newsletter, 1 we explored progress toward creating medicines that allow nerve cells to regrow. Here, we’ll explore another primary research goal, the development of medicines that induce the growth of new connections among the nerve cells in the brain.
The idea that a brain is comprised of interconnected nerve cells (‘neurons’) carrying small electrical currents was first proposed at the end of the 19th century.2,3 In the 1920s, it was first demonstrated that these electrical currents are transmitted between neurons by chemical substances (‘neurotransmitters’) acting in the minute space between neurons (‘synapses’).4 We now understand that ~600 trillion synaptic connections among 100 billion neurons create the brain’s function!5 Perception, learning, memory, thought, emotion, and movement result from neurotransmitters’ symphony of coordinated synaptic activation of neuronal networks.
Synaptic connections must be stable and durable to store memories and learning. But synapses must also be dynamic, strengthening some connections while weakening others in response to new experiences. There is a critical balance between stability and so-called ‘plasticity,’ the capacity of synapses to grow and change. During some phases of development, the organism is most advantaged by extreme plasticity; early in life, the brain’s interconnections must rapidly and continuously evolve. Later, it is advantageous that synaptic structures become more durable. It is, in fact, harder to ‘teach an old dog new tricks’, since synaptic networks become less flexible with age. The genetic programs that control synaptic activity determine the neuronal network’s relative degree of stability or plasticity. Maintaining a balance between stability and plasticity is critical for healthy brain function. Plasticity is needed for learning, and stability is required to retain the benefits of new learning.
In recent years, there has been a growing understanding of the normal regulation of synaptic formation, the role of specific neurotransmitters in synaptic function, and the impact of various classes of drugs on the formation of new synapses.6 Drugs affecting the synapse may modify the release or uptake of specific neurotransmitters or alter the activity of genetic systems that control the production or degradation of neurotransmitters. Many different neurotransmitters have been identified, and many other drugs have been identified which alter neurotransmitters or modify the formation of synapses and, as a result, change synaptic function.
A significant acquired brain injury that results in loss of function has disrupted both neurons and the synaptic connections between them. Severe injuries create conditions in brain tissue that disrupt synaptic activity in many ways immediately after injury and over the months and years after the acute event.7,8 Injured tissue can release substances that can cause overactivity of synapses, the disruption of pre-existing synapses, or a reduction in the ability to create new synapses.
For functional recovery to occur after a severe brain injury, new synapses must be created as other brain regions are recruited to compensate for lost function. There is now a sufficiently detailed understanding of the regulation of synaptic neurotransmission to permit the development of drugs that stimulate the formation of new synapses (“synaptogenesis”) after a significant brain injury. Recent studies indicate it is possible to stimulate the creation of new synapses in the brain.9,10 Several studies are underway to explore the value of drug-induced synaptic plasticity in promoting functional recovery after a severe brain injury. Other investigators have directly modified gene transcription to upregulate synaptogenesis.11 As the genomic and transcriptional controls of synaptogenesis are further clarified, investigators will inevitably seek to directly upregulate the formation of new synapses and, thereby, induce functional recovery after severe brain injury. The DLF views these lines of inquiry as promising and has, accordingly, prioritized the stimulation of synaptogenesis as a core strategy of brain regeneration research.
Another strategy to restore function after a brain injury is to replace lost neurons by either stimulating new neuronal proliferation or by transplanting cells that can become neurons in the recipient’s brain.12 If new neurons or neuronal precursors are introduced into an injured brain, it will be necessary to create a microenvironment that optimizes the formation of new synapses in response to intensive retraining. Some of the same drugs that stimulate synaptic plasticity may also be essential to condition the brain as it seeks to incorporate new neurons into damaged tissues. More to come about this research frontier in a future DLF newsletter.
References
- Dan Lewis Foundation Newsletter, Fall 2022.
- Scheuerlein, H., Henschke, F. & Köckerling, F. Wilhelm von Waldeyer-Hartz—A Great Forefather: His Contributions to Anatomy with Particular Attention to ‘His’ Fascia. Frontiers in Surgery 4, (2017).
- López-Muñoz, F., Boya, J. & Alamo, C. Neuron theory, the cornerstone of neuroscience, on the centenary of the Nobel Prize award to Santiago Ramón y Cajal. Brain Res. Bull. 70, 391–405 (2006).
- York, G. K., Iii. OTTO LOEWI: DREAM INSPIRES A NOBEL-WINNING EXPERIMENT ON NEUROTRANSMISSION. Neurology Today 4, 54 (2004).
- Wanner, M. 600 trillion synapses and Alzheimers disease. The Jackson Laboratory https://www.jax.org/news-and-insights/jax-blog/2018/december/600-trillion-synapses-and-alzheimers-disease.
- Kozorovitskiy, Y., Peixoto, R., Wang, W., Saunders, A. & Sabatini, B. L. Neuromodulation of excitatory synaptogenesis in striatal development. Elife 4, (2015).
- Jamjoom, A. A. B., Rhodes, J., Andrews, P. J. D. & Grant, S. G. N. The synapse in traumatic brain injury. Brain 144, 18–31 (2021).
- Merlo, L. et al. Alteration in synaptic junction proteins following traumatic brain injury. J. Neurotrauma 31, 1375–1385 (2014).
- Ng, S. Y. & Lee, A. Y. W. Traumatic Brain Injuries: Pathophysiology and Potential Therapeutic Targets. Front. Cell. Neurosci. 13, 528 (2019).
- Burlingham, S. R. et al. Induction of synapse formation by de novo neurotransmitter synthesis. Nat. Commun. 13, 3060 (2022).
- Sahin, G. S. et al. Leptin stimulates synaptogenesis in hippocampal neurons via KLF4 and SOCS3 inhibition of STAT3 signaling. Mol. Cell. Neurosci. 106, 103500 (2020).
- Xiong, L.-L. et al. Neural Stem Cell Transplantation Promotes Functional Recovery from Traumatic Brain Injury via Brain Derived Neurotrophic Factor-Mediated Neuroplasticity. Mol. Neurobiol. 55, 2696–2711 (2018).
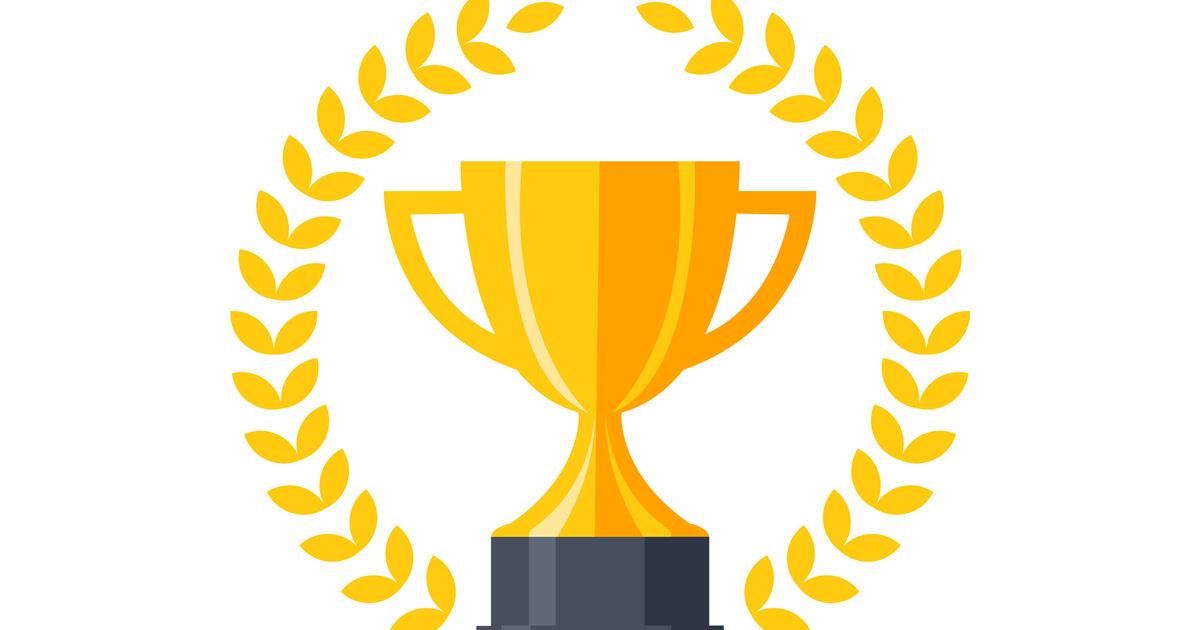
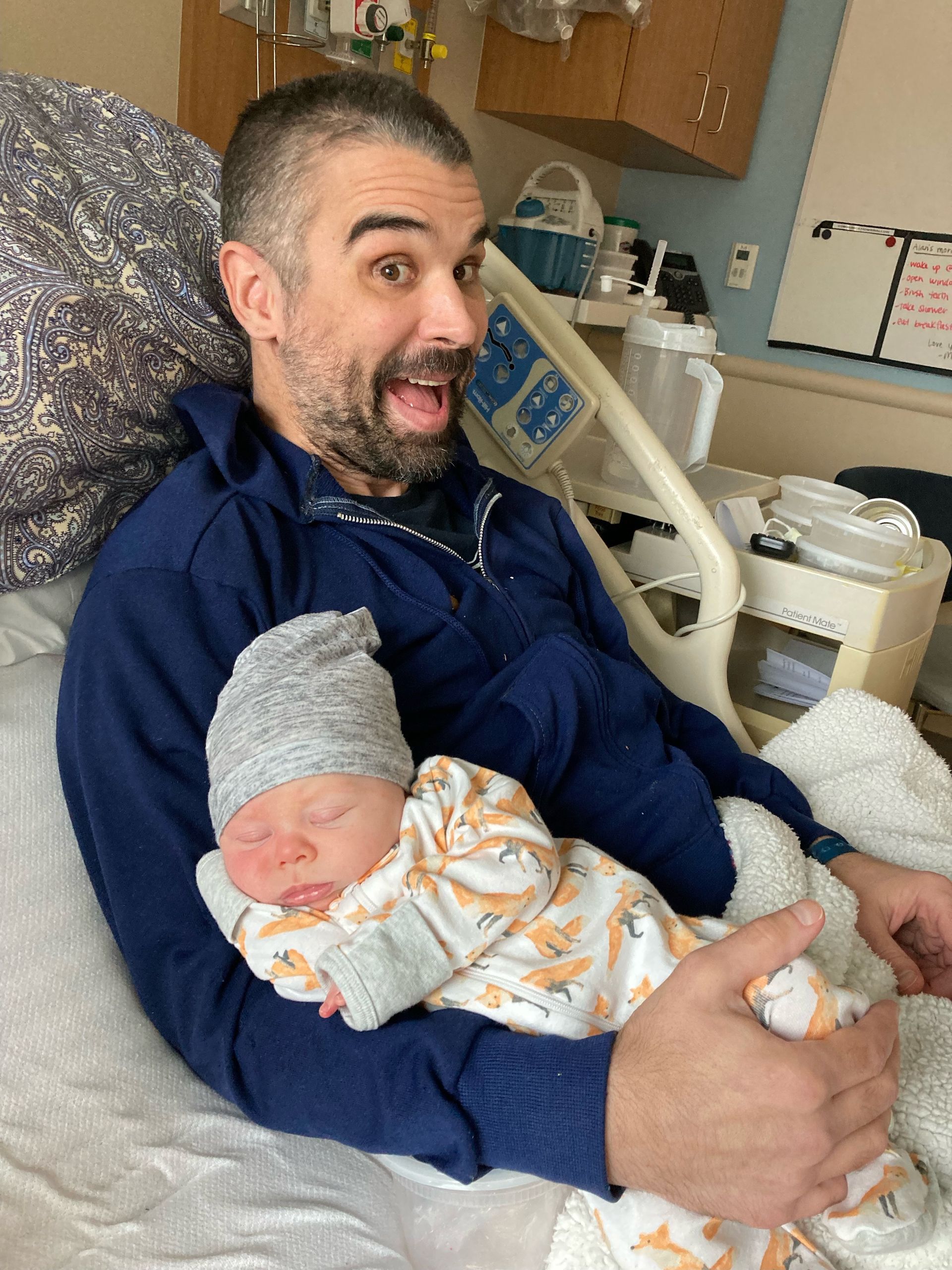