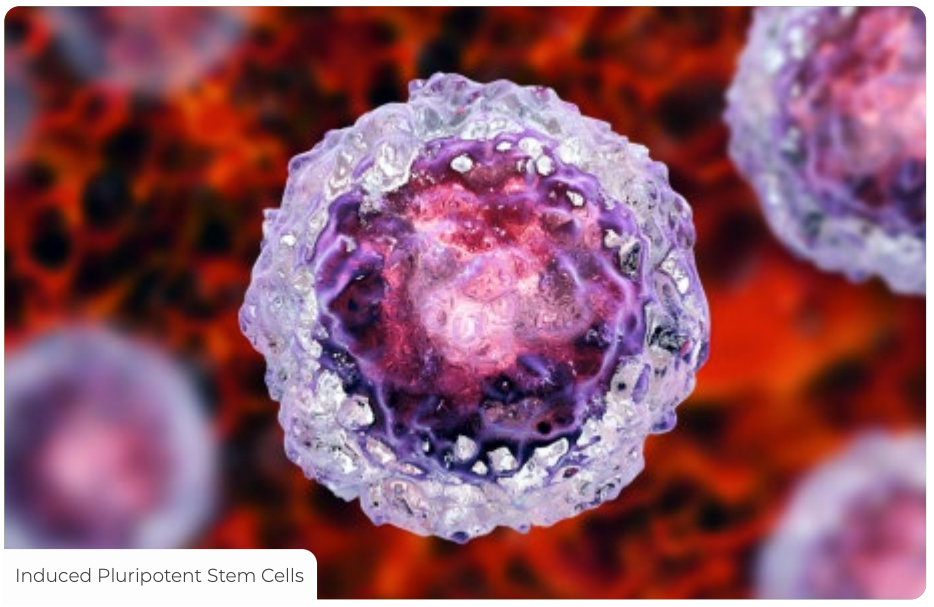
The idea of replacing damaged brain tissue is intriguing and, at the same time, seemingly implausible. When vital brain tissue is damaged or destroyed, the function served by this tissue is lost. Other brain regions may provide some functional compensation, but in adults, this compensatory adaptation is limited. The field of brain regeneration research is driven by the vision that a meaningful regrowth or regeneration of brain tissue might be achieved.
Embryonic cells know how to grow and form brain tissue. These cells contain all the information required to divide, migrate, connect, and differentiate into specific functional brain regions or subunits. Can this genetic knowledge somehow be unlocked or reactivated in a damaged, mature brain?
In recent decades, scientists have taken steps towards this goal. For example, scientists learned how to cause the fibroblast cells in a snippet of skin to revert to embryo-like cells, called induced pluripotent stem cells ('iPSCs'). These iPSCs have all the information necessary to form any mature tissue or organ. Under the right conditions, these cells can be guided to differentiate into nerve tissue, cardiac tissue, and many other mature structures.
Induced Pluripotent Stem Cells
Brain regeneration researchers have begun to seriously explore the possibility that cells or tissues derived from these 'reawakened' cells might be reintroduced into a damaged brain. 1 The hope is that these cells might engraft into the brain, recreate the damaged anatomy, and reconnect with other brain regions. This embedded patch might, in this scenario, be retrained, enabling the re-acquisition of the lost functions.
There are now reports of 'derived neurons' being introduced into a damaged brain to repair a damaged brain structure. One such example involves using iPSCs to generate dopamine-producing neurons, which are the type of neurons that degenerate in Parkinson's disease. In theory, these derived neurons can be transplanted into patients' brains to replace the lost neurons and restore dopamine function.
In one study, researchers transplanted iPSC-derived dopamine neurons into the brains of monkeys with a Parkinson's-like condition. 2 The transplanted neurons survived, integrated into the host brain, and produced dopamine, improving motor function. Clinical trials are underway to determine if this approach can be safe and effective in humans, with some encouraging initial results in both motor function and quality of life. 3 This highly targeted cellular replacement research is a long way from replacing large regions of the brain cortex lost from trauma. Still, its success demonstrates that, in principle, new cellular material derived from induced pluripotent stem cells can be used to replace lost brain functions.
Organoids
Several years ago, some teams observed that if iPSCs are cultured for a longer period under particular conditions, they begin to clump into tissues. The tissue aggregates that form from derived neurons begin to take on some of the forms and structures of a developing brain. These cellular aggregates are called
organoids. These brain organoids contain multiple cell types and regions found in the developing brain, including neurons, astrocytes, and oligodendrocytes. Because brain organoids resemble a developing brain, they are being used to study normal brain development and disease mechanisms.
4
Organoids possess the ability to self-organize in a dramatic fashion. The resulting structures manifest many features of developing brains. This demonstrates that the interplay between genomic instructions inherent in derived neurons and different forms of cell-cell signaling is sufficient to guide the formation of complex structures.
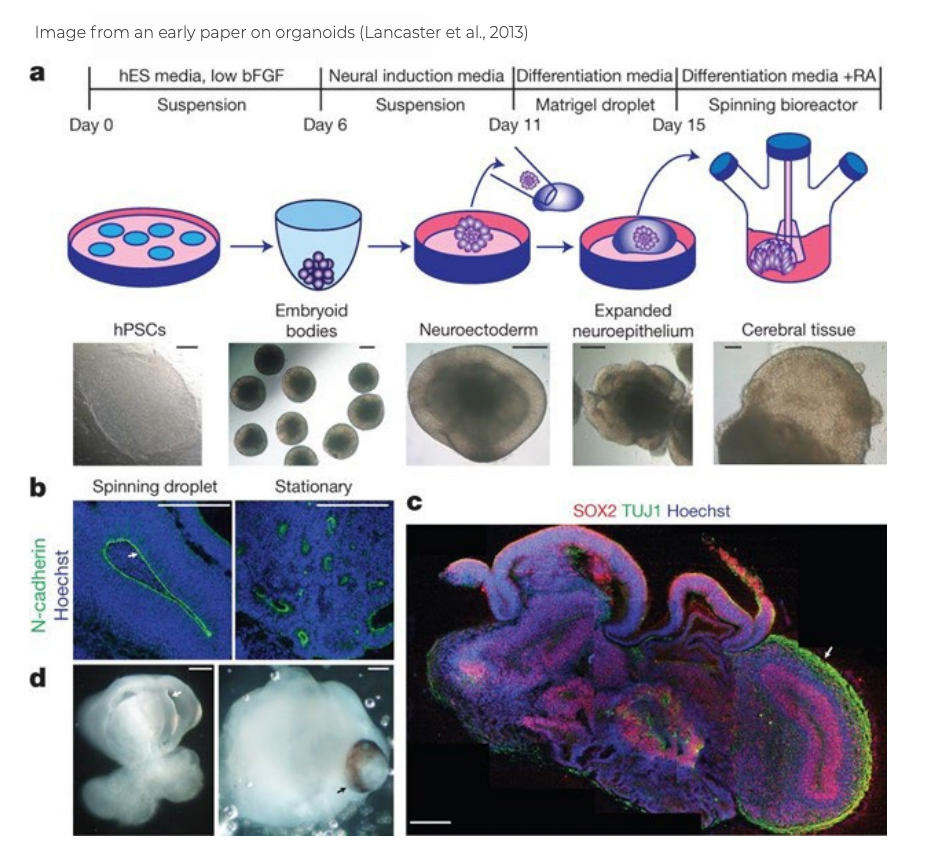
While there is a long path ahead to determine if human organoids can ever replace and replete brain tissue that has been destroyed or damaged, there has been important progress toward this goal in recent years.
In a study published in 2018, a team led by Dr. Fred Gage transplanted human brain organoids into the brains of adult mice.5 The study shows that organoid grafts developed into functional neuronal networks with axons that grew to multiple regions of the host brain. The grafts also had functional blood vessels and integrated microglia. They also showed that the grafts had neuronal activity and suggested that there was functional synaptic connectivity between the graft and the host brain. This study demonstrates that a human organoid can survive transplantation, grow, and create connections to an adult mouse brain.
In a more recent study, researchers transplanted human brain organoids into the brains of neonatal mice. They found that the transplanted organoids survived and extended projections over a significant distance to connect with the host brain. The transplanted human neurons could function and deeply integrate into the mouse neural circuits. Furthermore, the mice transplanted with cerebral organoids showed an increase in the startle fear response, suggesting that the organoids have the potential to modulate behavior.6 This study demonstrates that the connections formed between the organoid graft and the developing mouse brain are functionally active.
Two new studies demonstrate that the circuits created between the organoid and the host brain can respond to precise sensory stimuli and be trained to influence the animal's behavior. That is, a sensation perceived in the engrafted cells could be linked to a reward such that the animal changed its behavior after stimulation. The organoid in these studies becomes rewired into both the sensory and motivational circuits of the host. 7,8
While these and other studies9 provide some evidence that brain organoids could potentially be used to treat neurological disorders in the future, there remain many scientific, technical, and ethical barriers to the success of this approach. It is still very unclear whether human brain organoids can eventually be implanted to induce functional recovery in persons who have suffered a serious brain injury.
All cautions notwithstanding, the demonstration that a small snippet of skin contains the capacity to be transformed into pluripotent stem cells, matured into derived neurons, and then nurtured into a 'mini-brain' that can be implanted into another species, grow, and functionally integrate into that animal's brain has to be viewed as miraculous. This research is a source of great hope that meaningful functional recovery after severe brain injuries may be achieved.
References
- Chen, C., Kim, W.-Y. & Jiang, P. Humanized neuronal chimeric mouse brain generated by neonatally engrafted human iPSC-derived primitive neural progenitor cells. JCI Insight 1, e88632 (2016).
- Kikuchi, T.et al.Human iPS cell-derived dopaminergic neurons function in a primate Parkinson’s disease model.Nature548, 592–596 (2017).
- Doi, D.et al. Pre-clinical study of induced pluripotent stem cell-derived dopaminergic progenitor cells for Parkinson’s disease.Nat. Commun.11, 3369 (2020).
- Trujillo, C. A. & Muotri, A. R. Brain Organoids and the Study of Neurodevelopment.Trends Mol. Med.24, 982–990 (2018).
- Mansour, A. A.et al. An in vivo model of functional and vascularized human brain organoids.Nat. Biotechnol.36, 432–441 (2018).
- Dong, X.et al. Human cerebral organoids establish subcortical projections in the mouse brain after transplantation.Mol. Psychiatry26, 2964–2976 (2021).
- Wilson, M. N.et al.Multimodal monitoring of human cortical organoids implanted in mice reveal functional connection with visual cortex.Nat. Commun.13, 7945 (2022).
- Revah, O.et al.Maturation and circuit integration of transplanted human cortical organoids.Nature 610, 319–326 (2022).
- Ramirez, S.et al. Modeling Traumatic Brain Injury in Human Cerebral Organoids.Cells 10, (2021).
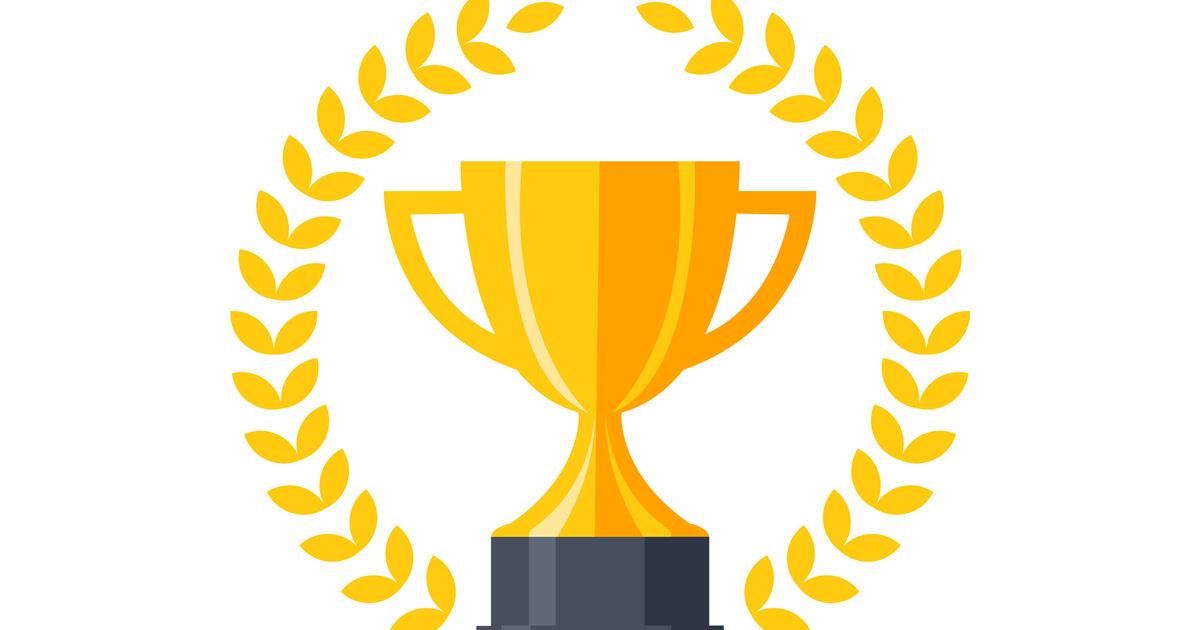
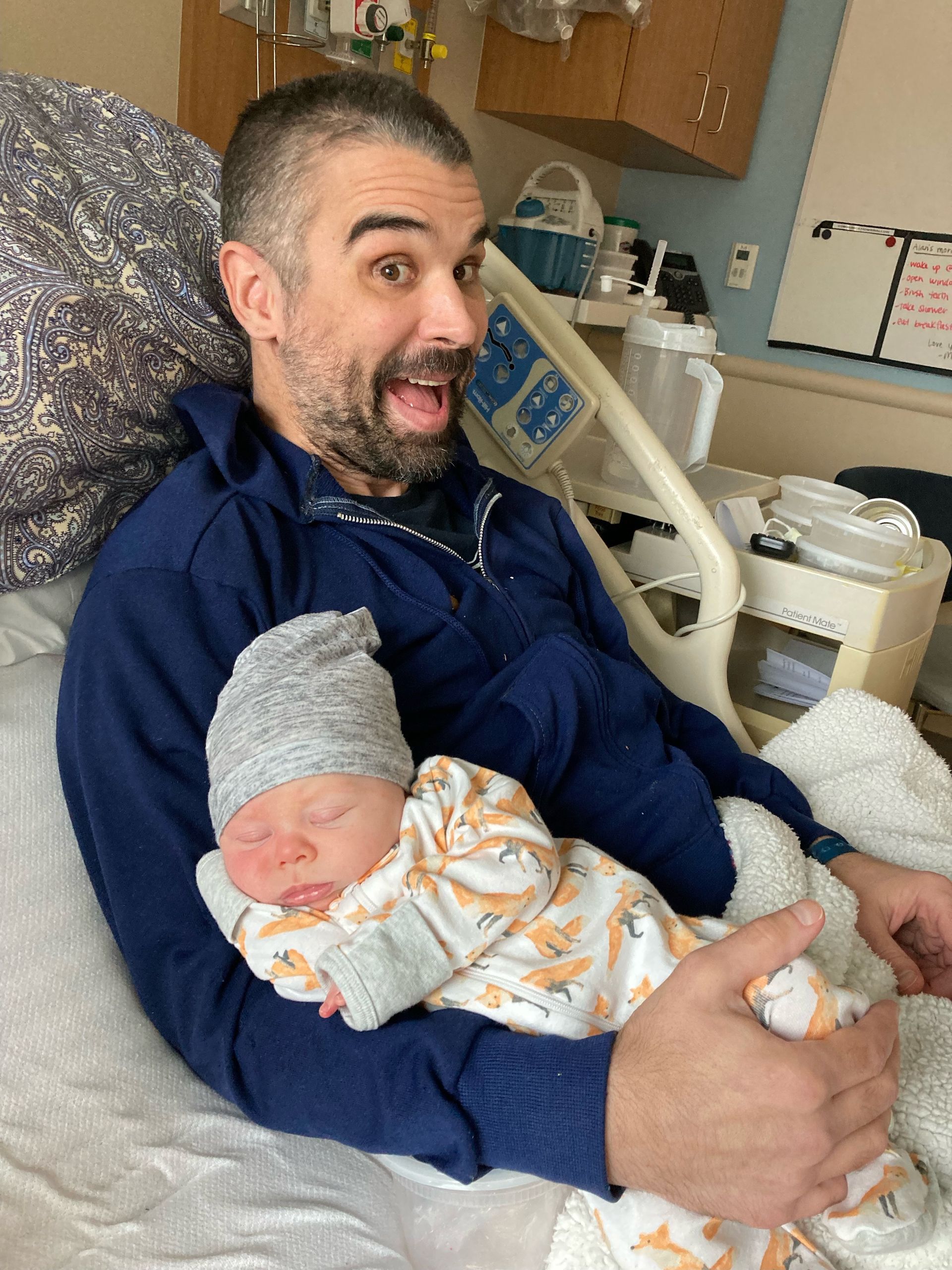